Brain Blast: When Jimmy Neutron Stars Collide
LIGO, or the Laser Interferometer Gravitational-Wave Observatory, in Livingston, Louisiana, made a splash in February of 2016 with its discovery that confirmed Einstein’s theoretical gravitational waves. Since then, the existence of these waves has been continually reaffirmed by LIGO through their detection of the same phenomena that was the subject of the breakthrough in 2016: the collision of black holes. On October 16th 2017, shortly after three team members were awarded the Nobel Prize in physics for their work with gravitational waves, LIGO released a paper detailing a collision of two neutron stars.
Previously observed gravitational waves were the product of black hole collisions — not much there visually — but LIGOs most recent observation on August 17th was a neutron star collision. With optical data to accompany LIGOs record of the gravitational wave event, future research to reconcile Newtonian and quantum physics may gain a more informed direction. Working to find a unified field theory — a way to consolidate quantum physics and classical physics — has been the subject of theoretical and applied research since Einstein’s heyday.
On August 17th, both the LIGO in Livingston and its recently constructed sister observatory Virgo in Pisa, Italy detected a signal unlike any observed before. Signals of black hole mergers typically last fractions of a second, but the one in question lasted well over 30 seconds.
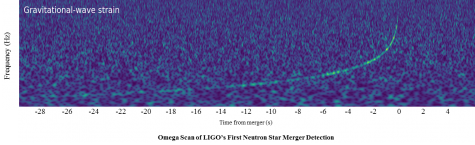
One point seven seconds after this detection — and the ensuing chaos over a potentially new event observation — the Fermi Gamma-ray space telescope recorded a short gamma ray burst. Gamma ray bursts are frequent events so this would usually be inconsequential, but given the LIGO data it seemed likely that the burst came from the collision detected. For the first time the waves observed at LIGO were linked to an observation of electromagnetic radiation, which could help direct future research both gravitationally and cosmologically.
As for those neutron stars — they’re a very special sort of degenerate star. When a star has degenerated, it can no longer perform nuclear fusion at its core (meaning it has burned up all of the atomic fuel inside of it). The degeneration process is complex and star-specific, but what’s most important is that the heaviest element which can be created at the core of any given active star is iron.
But wait, you may think smartly to your scholarly self, iron is like, number twenty-something out of one-hundred-and-something elements, where did the rest of those dudes come from? Well, good question! Heavier elements may come from collisions of those neutron stars that I called special earlier without explanation. What separates neutron stars from other, lamer, degenerate stars is their density.
The larger a star is, the more mass and gravity it has. When a star between four and eight solar masses — between four and eight times the mass of the sun –collapses, it typically forms a neutron star. An average neutron star radius is somewhere around twenty kilometers, but their masses can equal up to one and a half times that of the Sun. They are so dense, the electrons and protons that make up the star are smooshed together forming neutrons — hence the name. Neutron stars start out very large, between eight and four solar masses, and rapidly shrink, to less than or equal to one and a half solar masses, so they rotate at a hilariously high rate due to the conservation of angular momentum. What these stars undergo is the cosmic equivalent of spinning in an office chair, legs extended, and then pulling them in.
The collision of two of these highly energetic objects, each with huge amounts of gravity due to their density, is likely able to produce elements heavier than iron, like gold, silver, etc. that a single star is unable to make.
This sort of collaborative discovery has been in the works as long as LIGO has been functioning; they’ve signed agreements with ninety other astronomical observatories in the hopes of documenting any electromagnetic radiation produced by a gravitational wave event. Once the data from LIGO, Virgo, and the Fermi telescope were distributed to their astronomy partners, all eyes were on the section of the sky predicted by the LIGO team to be the location of the event. Within twelve hours, partners of LIGO who had received the coordinates found and imaged the aftermath of the collision — in a galaxy around 130 million light years away.
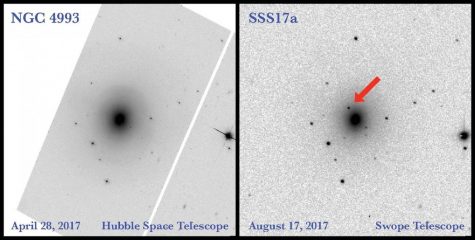
The EM counterpart to a gravitational wave detection was finally observed, it was confirmed that merging neutron stars produce short gamma ray bursts, and it is very likely that neutron star collisions produce heavy metals like gold and silver.
Care for yet another slice of this sweet, sweet science pie? The distance to the galaxy wherein the collision took place, around 130 million light years, was consistent with the distance estimated by LIGO based solely on the gravitational waves observed. Which means that LIGOs methods for determining the distance to the source of a gravitational wave event is seemingly accurate.
High octane events like this observation and subsequent discoveries keep interest in fields like physics, astronomy, astrophysics, and even engineering, peaked. While lack of interest in STEM isn’t an issue in the Bay Area, super-dense dead stars slamming into one another and spewing gold everywhere encourages children to perhaps reach for the moon rather than for ole Elon Musk.